Featured image: The earliest examples of life on Earth are microbial buildups known as stromatolites, like these 1.8 Ga old examples from Great Slave Lake, Canada. What changed on our planet for organisms to evolve from microbes to macroscopic lifeforms?
Paper: Ediacaran reorganization of the marine phosphorus cycle
Authors: Laakso, T.A., Sperling, E.A., Johnston, D.T., and Knoll, A.H.
This is a guest post by Akshay Mehra and Danielle Santiago Ramos. Contact us to submit a guest post of your own!
The history of life on Earth—as recorded in the rock record—stretches back to more than 3.5 billion years ago (Ga). The earliest fossilized remains of living organisms appear in the form of stromatolites, which are laminated constructions built in part (or completely) by microbes. While there have been some tantalizing hints that living organisms were mobile by 2.1 Ga (Albani et al., 2019) and multicellular by 1.6 Ga (Bengston et al. 2017), what is definitively known is that by ~750 million years ago (Ma), complex microscopic lifeforms were widespread on our planet. As time progressed, life became macroscopic. Then, during the Cambrian Era (beginning 539 Ma), most modern phyla (i.e. a grouping of organisms based on body plans) appeared in a flurry of diversification so drastic that it has been nicknamed “the Cambrian explosion.” Scientists are still trying to understand what combination of physical and biological processes may have driven the Cambrian explosion.
In a recently published paper, Laakso et al. use geological observations and computer models to suggest that a change in the way the element phosphorus (P) was delivered, consumed, and stored in Earth’s oceans around the Ediacaran Era (which began ~635 Ma and lasted until the start of the Cambrian) ended up completely reorganizing our planet’s biogeochemical cycles. This change brought about the world as we know it today. The authors focus on P because it is a globally limiting nutrient, meaning that the growth of life is constrained by the amount of P in the ocean. In their paper, the authors consider what happens to P as it is liberated from rocks through weathering, delivered to the oceans, then used by living organisms, and finally either stored in sediments on the ocean floor, or, if the sinking bodies of dead organisms are consumed, released back into the water column (in a process known as remineralization).
To start, Laakso et al. point out that while phosphorite deposits (essentially, rocks with large amounts of P) can be found in pre-Ediacaran rocks, their size and extent increase dramatically around 600 Ma. The authors write that this signal is the result of a change in the P cycle on Earth rather than a preservation bias (i.e., older rocks being less well preserved than newer ones). Importantly, this transformation appears to coincide with other major biogeochemical shifts on Earth, including the rise of macroscopic organisms. But what was the cause of the increase in phosphorite deposits? The authors point out that the rate of P delivery to shallow ocean environments—where it may be locked in sediments—is what controls the formation of P-bearing minerals. Previous researchers suggested that mountain-building events exposed P-rich rocks to weathering, which, in turn, ended up boosting the rate of P delivery to the oceans. However, Laakso et al. stress that such a mechanism would only create a short-lived pulse of phosphorus to the oceans, and therefore cannot explain the persistent change observed in the rock record.
Instead, the authors link the increase in the rate of P delivery to changes in P remineralization. Essentially, if the amount of sinking material in the oceans (or the amount of oxidants, such as sulfate or oxygen) go up, then more matter is remineralized, thereby boosting the delivery of P to shallower waters. Laakso et al. suggest that it was this process—specifically with the amount of oxidants in deep waters increasing—that occurred during the Ediacaran. As the amount of P went up in shallow waters, primary productivity also increased. And since more living organisms means more sinking dead bodies, more material was delivered to the deep ocean to be remineralized (thereby making the effects even stronger, or creating a positive feedback loop). A crucial result of higher primary productivity was the increase in the concentration of oxygen in the ocean-atmosphere system, a condition necessary to sustain macroscopic life.
Having presented their model, the authors grapple with an important question: since the ocean was largely depleted in oxygen during this time, which oxidants were responsible for increased deep-water remineralization? Here is where mountain-building events and continental weathering come into play. Laakso et al. point out that seawater sulfate concentrations increased at the same time as Ediacaran phosphorites, as evidenced by the rock record of evaporites. As an important product of continental weathering, sulfate (SO42-) can be consumed by so-called sulfate reducing bacteria. During consumption, organic matter is remineralized. Since the remineralization of organic matter is greater in water masses with larger concentrations of sulfate, it stands to reason that increased sulfate in Ediacaran oceans may have led to more P remineralization in the water column. P delivery to surface waters would then increase primary productivity and oxygen production, thus leading to even more organic matter remineralization.
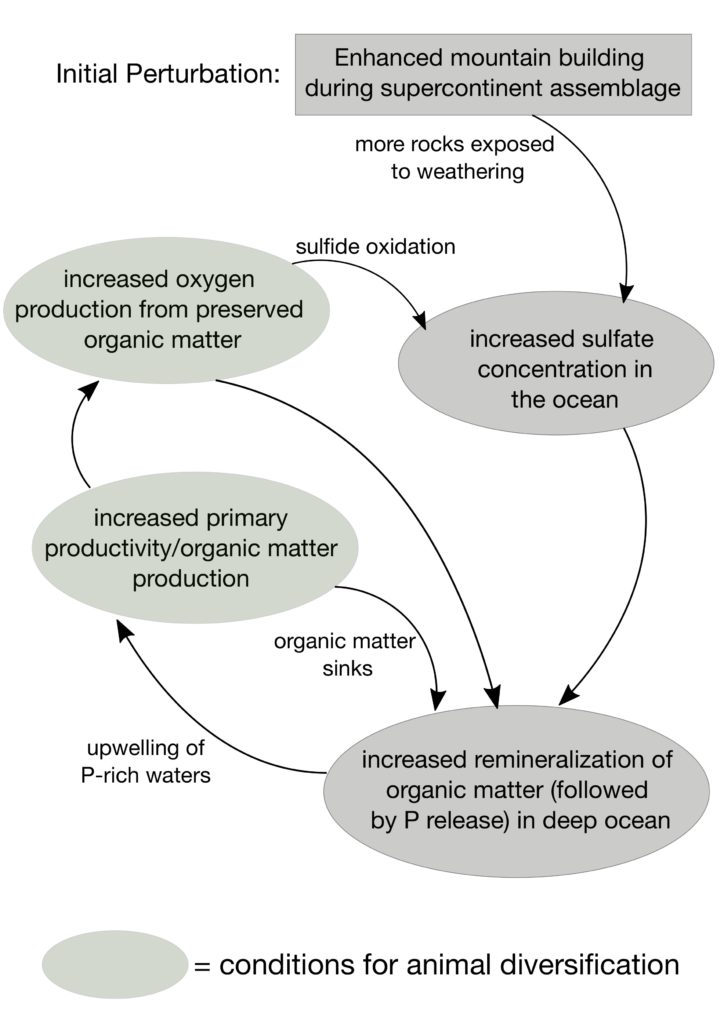
The important contribution of Laakso et al. is to recognize that existing models of animal diversification fall short when explaining persistent, step-change transformations observed in the Ediacaran. Using the same initial trigger as previous models, Laakso et al. unravel a series of changes that brought about the conditions necessary for animal diversification, including a more abundant food supply (from P fertilization of the shallow ocean) and increased oxygen supply (from higher organic matter production). The authors’ hypothesis, involving feedbacks between mountain-building events, ocean redox chemistry, and the biogeochemical cycling of phosphorus, elegantly bridges an existing theoretical gap.
References
El Albani, A., et al. “Organism motility in an oxygenated shallow-marine environment 2.1 billion years ago.” Proceedings of the National Academy of Sciences 116.9 (2019): 3431-3436.
Bengtson, S., Sallstedt, T., Belivanova, V., Whitehouse, M. “Three-dimensional preservation of cellular and subcellular structures suggests 1.6 billion-year-old crown-group red algae.” PLoS Biology 15.3 (2017): e2000735.
Squire, R.J., Campbell, I.H., Allen, C. M., Wilson, C.J.L. Did the Transgondwanan Supermountain trigger the explosive radiation of animals on Earth? Earth Planet. Sci. Lett. 250, 116–133 (2006).
Did a change in phosphorus cycling lead to the diversification of macroscopic life? by Akshay Mehra and Danielle Santiago Ramos is licensed under CC BY-SA 4.0