Authors: David De Vleeschower, Anna Joy Drury, Maximilian Vahlenkamp, Fiona Rochholz, Diederik Liebrand & Heiko Pälike
Featured image: Benthic foraminifera collected from the North Sea in 2011. Image courtesy of Hans Hillewaert, licensed under CC BY-SA 4.0
Faced with a rapidly warming world, we all have the same questions on our collective minds: how will climate change restructure Earth and what can we do to adapt to those changes? One thing we do know is that the climate is intimately connected to the carbon cycle. When large amounts of carbon get moved between reservoirs (on land and in the ocean and atmosphere), changes in climate ensue. Currently, carbon stored on land is being moved to the atmosphere through anthropogenic CO2 emissions, causing global warming and its various cascading effects. What’s more, looking back in Earth’s history, researchers have established that moving carbon from the atmosphere to the ocean, or back onto land, has had a cooling effect. Just this past year, researchers from the University of Southampton investigated several factors affecting past carbon-climate connections, offering new understandings that could help address climate action moving forward.
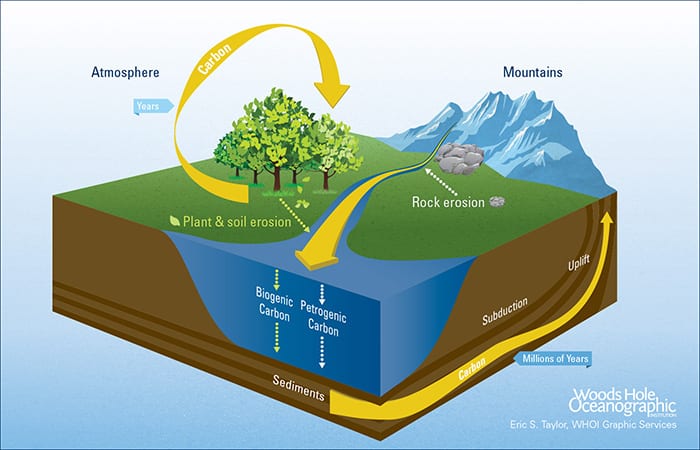
Simplified carbon cycle diagram, showing how carbon moves between major reservoirs (land, atmosphere, deep ocean). Image created by Eris S. Taylor of the Woods Hole Oceanographic Institution.
The recent study by De Vleeschouwer et al. explored the relationship between climate and the carbon cycle by compiling records of stable isotopes of carbon and oxygen from 35 million years ago to the present, observing their interaction through time. Since we can’t directly measure temperature or atmospheric CO2 back in time, researchers make use of paleo-proxies, surrogate data for environmental conditions. Proxies come from archives like ice-cores, tree rings, and deep ocean sediments, acting as nature’s history books—recording information on how the Earth has changed over time, when and where it’s gotten colder or warmer, wetter or drier, and more or less hospitable to different forms of life.
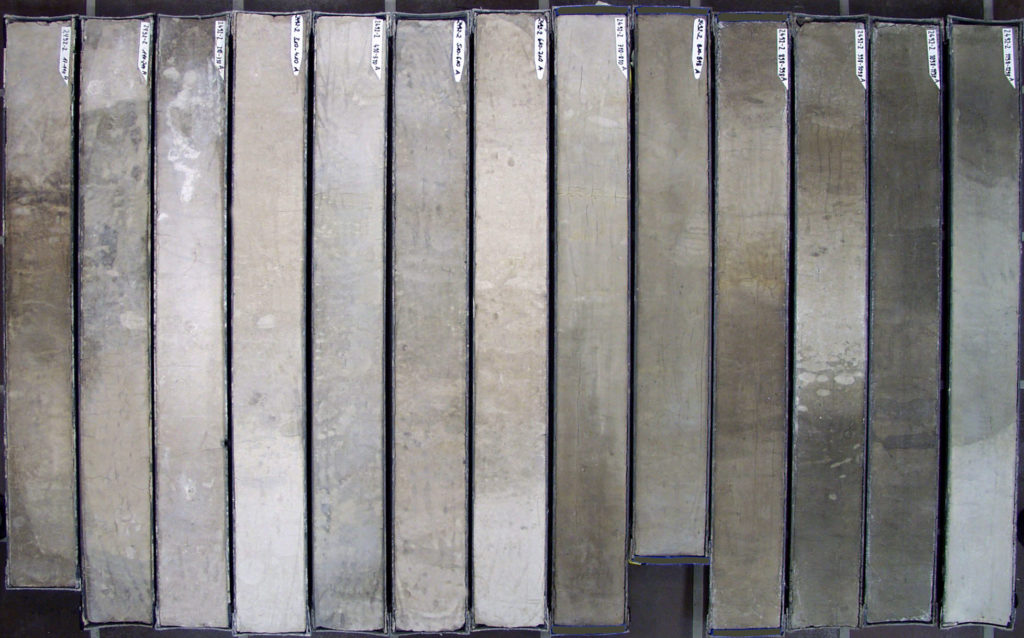
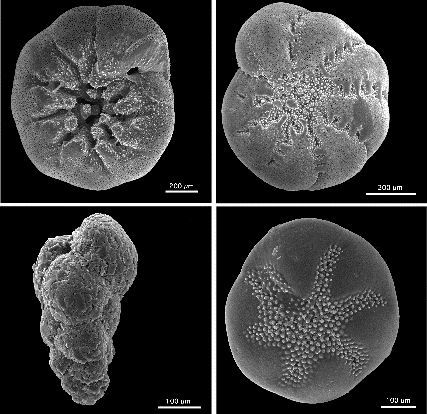
Top: Deep ocean sediment cores whose light/dark colors indicate climatic changes. Image courtesy of Hannes Grobe, licensed under CC-BY 3.0. Bottom: Several species of benthic foraminifera shells from deep sea sediment cores. Image courtesy of USGS, Public Domain.
The De Vleeschouwer et al. study used deep ocean sediments and the tiny shells of long-deceased, single-cellular organisms called foraminifera within them to obtain their proxy data—the stable isotopes. As the researchers explain, the stable isotope ratio of oxygen-18 to oxygen-16 in the shells is a proxy for the climate system, recording both deep-ocean temperature and ice volume changes on land. The ocean’s stable isotope ratios of oxygen increase during colder times (with more land ice) and decrease during warmer times (with less land ice). Here’s how: oxygen-16 is lighter than oxygen-18, which means more of it can be picked up and carried away by evaporation while oxygen-18 mostly stays in the water. Once in the atmosphere, light oxygen-16 remains in the clouds while heavy oxygen-18 gets rained back down to Earth. By the time the clouds reach the polar regions, light oxygen-16 is about all that is left—making snow and ice extremely rich in oxygen-16 and depleted in oxygen-18. This creates a negative oxygen-18 to oxygen-16 ratio in snow/ice and a positive ratio in water. So, when climate is cold the ocean’s oxygen isotope ratio increases as oxygen-16 is taken out of the water and put into ice on land. Conversely, when climate warms and the ice melts, oxygen-16 is flushed back into the ocean, decreasing its oxygen isotope ratio.
Stable isotope ratios of carbon, a proxy for global carbon storage changes, are a bit more complicated. Carbon storage in the ocean can be affected by what’s occurring on land, in the atmosphere, or in the ocean itself, including changes in vegetation, precipitation, ocean mixing, biological productivity, and tectonic uplift. That’s a lot of interactions to keep track of, all which affect the ocean’s ratio of carbon-13 to carbon-12 differently. However, the authors stress that over longer periods of time (say, the 35 million years they studied) these changes in the carbon isotope record are overlaid by a stronger, global pattern.
By comparing the carbon and oxygen isotope records, De Vleeschouwer et al. aimed to tease out how the climate and carbon systems varied from 35 million years ago to the present, and whether they changed in the same ways over the same time periods. They found that the climate and carbon systems had been changing in sync from 34 to 6 million years ago: when carbon isotopes ratios increased, oxygen isotopes increased, and vice versa. After the 6-million-year mark, however, the carbon-climate relationship suddenly flipped, so that when stable isotopes of oxygen increased, stable isotopes of carbon decreased. This, they claimed, was suggestive of a reorganization of the carbon-climate system.
After analyzing the timing of the changes, the authors hypothesized that the flip in the carbon-climate relationship was due to Arctic cooling and the establishment of the Greenland ice sheet in the northern hemisphere. Previously, the only persistent ice sheet on the planet could be found on Antarctica. An ice-free northern hemisphere allowed for significant carbon storage in forests and peatland. However, the addition of an ice sheet in the northern hemisphere, coupled with the general cooling of the Late Miocene, led to a major decrease in the land’s ability to store carbon. Additional ice cover during glacial periods diminished the possibility for carbon storage on land even further by enveloping swaths of forested regions in ice. Large stores of carbon-12, once harbored in these forests, were moved into the ocean, lowering its overall isotopic ratio. In sum, this resulted in increased oxygen isotopic ratios (from the ice sheet), but decreased carbon isotopic values (from the loss of forests). Even when the ice retreated, the cold, dry climate favored grassier, tundra biomes which store much less carbon than the boreal forests that once grew in their place. This maintained the out-of-sync relationship of the carbon-climate system.
But as it turns out, even when the carbon-climate system was in sync from 35 to 6 million years, it wasn’t fully synchronous. De Vleeschouwer et al. observed leads and lags between the carbon-climate systems’ changes. They established that, though the climate system (oxygen isotope ratios) typically led changes in the carbon system (carbon isotope ratios), the lead-lag relationship could be reversed. This switch seemed to be brought about by an increase in weathering—the breakdown of rocks. When this occurred, changes in the carbon system (carbon isotope ratio) would lead—happen before—changes in the climate system (oxygen isotope ratio). Importantly, the authors found that the lead-lag switch was triggered by differences in the shape of Earth’s orbit at various points in history. When Earth’s orbit is more circular, both summers and winters are less extreme, while a more oval-shaped orbit causes more seasonal extremes of heat, cold, rain, and drought and therefore, more weathering.
According to the authors’ hypothesis, the carbon system lead (from 35 to 26 million years ago) was caused by a more circular orbit. This kept the climate cooler, and allowed Antarctic ice sheets to grow larger. These massive ice sheets contributed heavily to weathering and erosion by crushing, grinding, and scouring rocks. Weathering of this kind triggered the ocean to take CO2 from the atmosphere to help dissolve rock material that has made its way to the ocean. And in removing CO2 from the atmosphere, the climate continued to cool. Thus, the uptick in weathering caused changes in the carbon system, which then led to changes in the climate system.
This understanding of the lead-lag relationship only holds up until about 26 million years ago. After that, the modern monsoon system was established. This created a powerful new way to weather rocks. The authors observed that when the Earth’s orbit was more oval-shaped, the monsoon system intensified, producing more rain, fire, and drought. All of these weather extremes led to an increase in the weathering of rocks which, just as before, caused the ocean to take up CO2 and cool the climate. Here again, a carbon system change (taking up CO2 from the atmosphere) led to a climate system change (global cooling). In both of these periods of Earth’s history, the outcomes were the same—carbon changes caused climate changes. De Vleeschouwer et al. revealed that they were triggered by entirely different conditions in Earth’s orbital shape and weathering mechanisms.
It seems that in looking to the past with the modest hope of solidifying our understanding of the carbon-climate system relationship, we are instead reminded of how nuanced and complex it can be. The work of De Vleeschouwer et al. highlights the need for continued research directed toward developing a deep understanding of carbon-climate system interactions. This research, though rooted in studies of Earth’s past, provides critical insights that will guide our understanding of carbon, climate, and our future.
It’s complicated; deciphering mixed signals of the carbon-climate relationship in Earth’s past by Ingrid Izaguirre is licensed under CC BY-SA 4.0